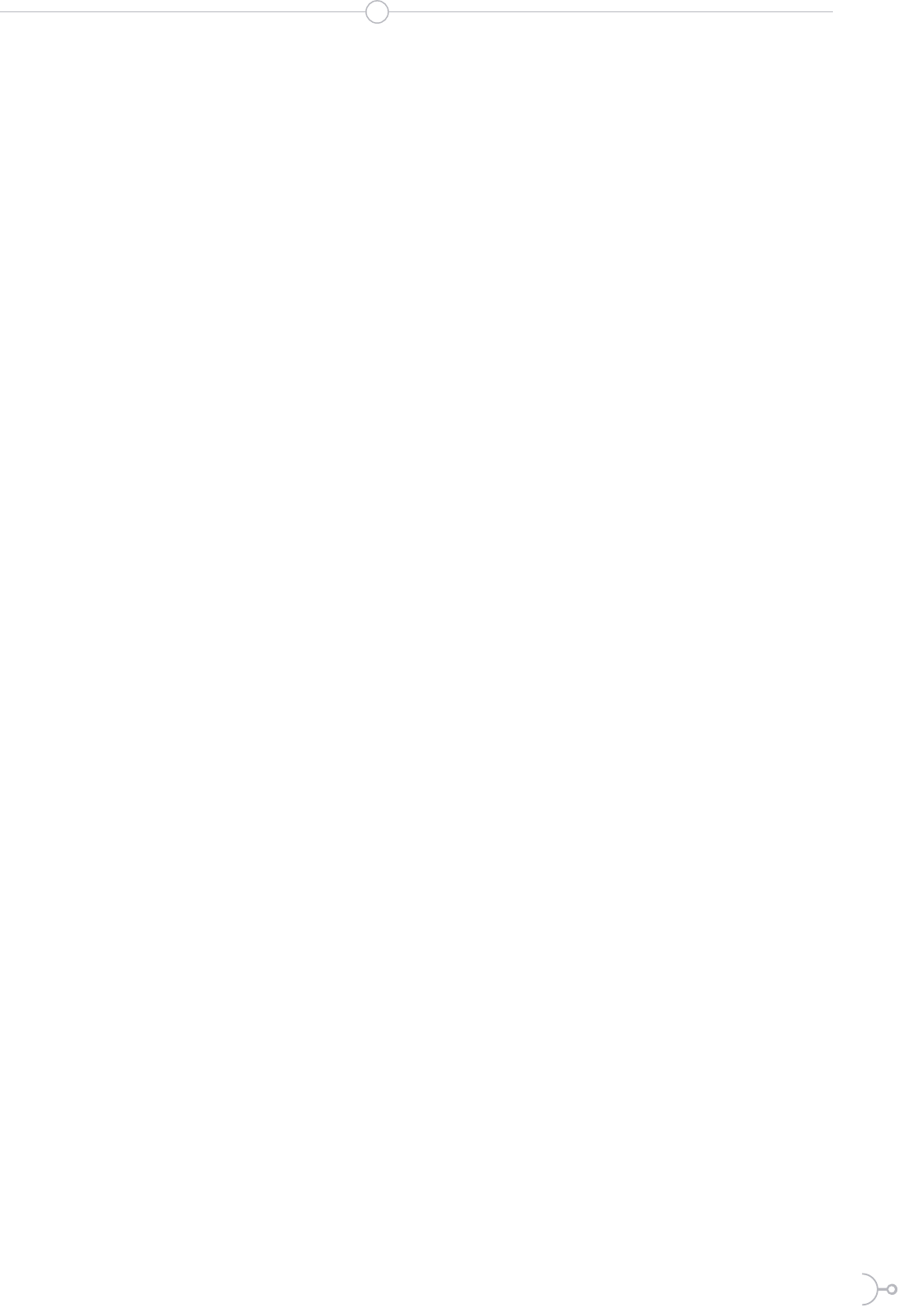
51
th Sector II (PARSALUD II), which is
the improvement of the 748 strategic
PHI through the development of Public
Investment Projects (PIP) with a time
horizon of 15 years (2). Each PHI has
roof areas potentially useful for the use
of radiant energy incident and to imple-
ment them with microgrids (MG) with
a good impact on the environment and
reduction of operational costs.
To analyze this case, a mathematical
model was constructed to estimate the
feasibility, return time and volumes of
production/consumption of electricity.
MG are small-scale, supply networks
designed to supply electrical and heat
loads for a small community. From a
grid point of view, the main advantage
of a MG is that it is treated as a contro-
lled entity within the power system. It
can be operated as a single aggregated
load. This ascertains its easy controllabi-
lity and compliance with grid rules and
regulations without hampering the relia-
bility and security of the power utility.
From a customers’ point of view, MGs
are benecial for locally meeting their
electrical/heat requirements. They can
supply uninterruptible power, improve
local reliability, reduce feeder losses
and provide local voltage support. From
a environmental point of view, MGs re-
duce environmental pollution and global
warming through utilization of low-car-
bon technology (3).
MG concept relates to a system which
coordinates locally the demand and su-
pply of energy. MG is essentially an
active distribution network because it
provides a platform for the integration
of various energy sources distributed
through a communications system that
allows control actions at distribution
voltage level (3). There are many pos-
sible congurations which may contain
generation of renewable and non-re-
newable electricity, storage and con-
trollable loads with priority categories
according to the user (4). Therefore, we
want to have: an MG with photovoltaic
(PV) plant; control, monitoring and supervision in auto-
nomous real time in PHI’s electric system and comply
with Peruvian legislation (5).
A MG is not an electrical conguration: (a) without a
load, (b) having only electrical charges, without micro-
sources, (c) without monitoring and control despite ha-
ving microsources, since their operation would not be
quantiable or optimized, (d) a conguration that has all
the elements, but insufcient carbon credits (6).
MATERIALS AND METHODS
About the PHI.
The Ofce of Investment Projects (IPO) of the Gene-
ral Ofce of Planning and Budget of the MOH by RD
No. 010-2012 / EF-68 approved the Minimum Contents
Specic 012 (MCS 012), a technical guidethat authori-
zes the use of renewable energy in PHI [2] but it does
not say how nor mentions modern trends as MG. MOH
by means of PARSALUD makes the evaluation of PHI
called “strategic” to improve their operational capacity
(7), This work is a contribution for the evaluation and
installation of MG in PHI of low electricity demand. The
PHI of Category I and II have usually a roof from 2,000
m
2
to 13,000 m
2
.
Solar Map of Peru.
Peru is politically constituted by 24 regions. A solar map
of Peru is available at (1). The largest PHI are located in
the capital of each region, therefore the solar radiation
in the capital of each regionis considered as a reference.
From (7) three values are assumed: Maximum (Rmax):
6.08 kWh/m
2
day; Average (Rave) 5.17 kWh/m
2
day, and
Minimum radiation (Rmin) 4.42 kWh/m
2
day.
Wind energy was not considered because installation
of wind turbines depends largely on the location; wind
speed varies during the year, depending on the season,
changes daily and have changes in short term (seconds
to minutes) both speed and direction (8). Currently there
exists a wind map of Peru (9) with only general informa-
tion; one needs to installation of measuring equipment in
PHI and needs real time records for an adequate technical
and economic evaluation of the wind resource (10).
PV Solar Panel Technology
There is a continuous improvement in materials and cons-
truction processes in solar panels (11). For this study, a
Jorge Luis Mírez Tarrillo